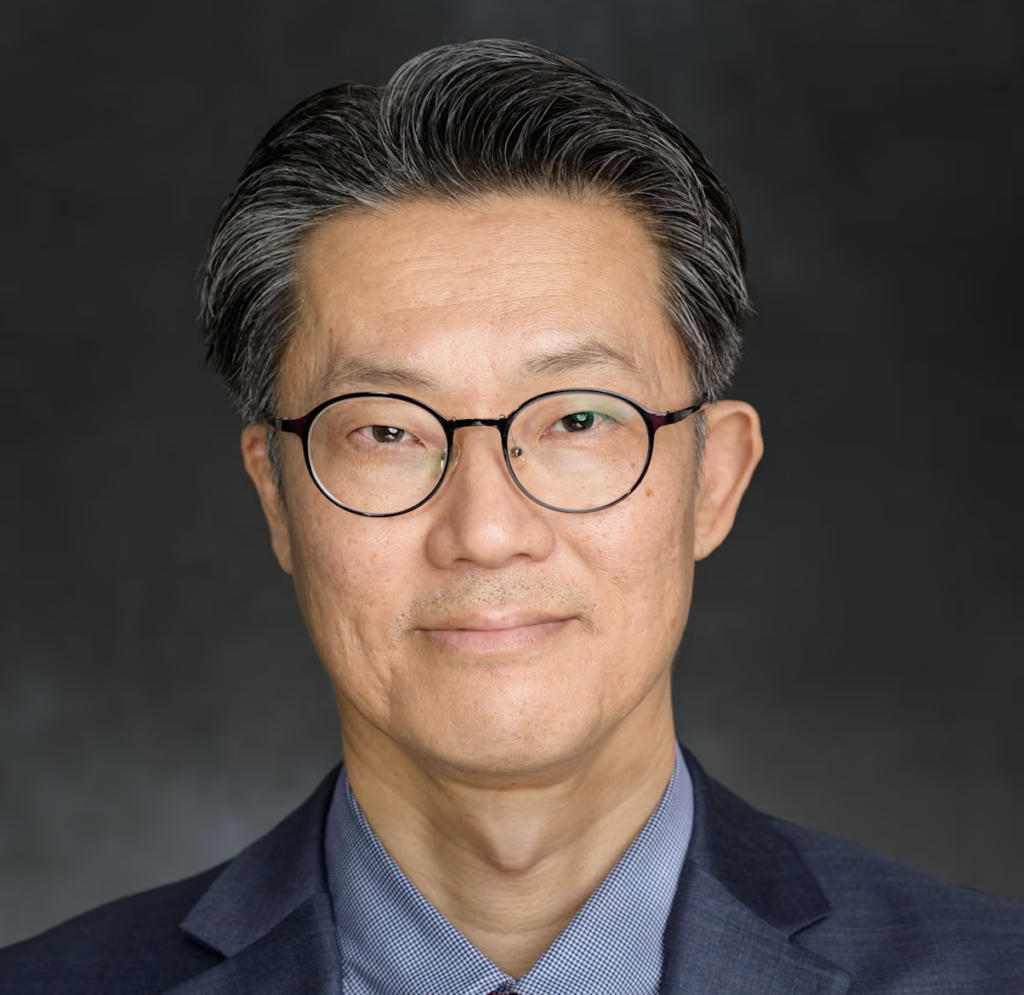
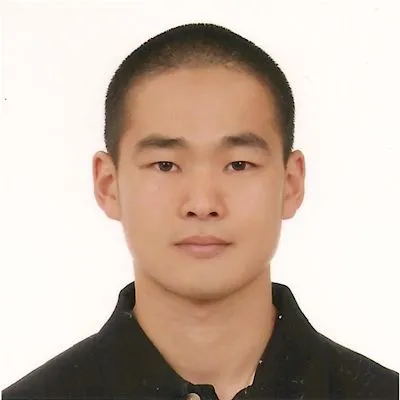
Photos courtesy of stevens.edu
At the forefront of groundbreaking research at Stevens, Dr. Rod Kim and Dr. E.H. Yang are tackling some of today’s most pressing technological challenges. Dr. Kim, an Assistant Professor in the Department of Electrical and Computer Engineering, is working to enhance communication reliability during spacecraft reentry, while Dr. Yang, a Professor in the Department of Mechanical Engineering, is exploring next-generation materials for more efficient electronic devices. Their studies push the boundaries of space technology, material science, and engineering, leading to advancements with many applications.
Reentry of a spacecraft is as stressful for the astronauts inside as it is for those on the ground working to ensure a safe landing. As the spacecraft plunges into the atmosphere, the rapid compression of air in front of it generates extreme heat and friction between gas molecules, creating a plasma layer. This layer releases free electrons into the flow field, disrupting signals and blocking “not only communication, but also navigation and data monitoring,” writes Stevens News. This phenomenon, known as radio blackout, poses a major challenge for spacecraft reentry. Dr. Rod Kim recently received a $550,000 National Science Foundation (NSF) CAREER Award for his study of “Versatile RF [Radio Frequency] Electronics for Extremely High-Temperature Sensing and Communications.” His team, including students from high school to the graduate level, aims to develop high-frequency electronics that can endure temperatures up to 1000 °C, reducing the risk of radio blackout.
Kim’s team is developing “high-frequency radio frequency electronics, novel materials, and measurement techniques that can be used even in these extreme plasma environments,” writes Stevens. His research focuses on 3D-printed ceramic and insulating materials capable of withstanding high temperatures while preserving their electromagnetic properties. These innovations include ceramic structures to combat heat-induced interference for accurate signal transmission, high-temperature sensors that adapt to changing electromagnetic properties for reliability, advanced signal trackers with on-chip processing to reduce noise and enhance real-time communication, and AI-powered ceramic fiber systems that dynamically adjust to temperature fluctuations for peak performance. Beyond advancing safer and more efficient space communication, Kim provides students opportunities to learn advanced circuitry and test equipment, building skills for future engineering careers. This technology also applies to “hypersonic defense systems, industrial monitoring, and other military and industrial uses that require flawless sensing and data transmission in extreme conditions.”
In spite of their remarkable capabilities, modern technologies often struggle with reliability, creating frustrations and limitations in our daily lives. Whether it is a cell phone constantly on low battery, a slow-performing laptop, or AI failing to process data, these challenges highlight the ongoing need for innovation that prioritizes both functionality and dependability. Dr. E.H. Yang and his PhD student Seiwein Chen are collaborating with researchers from Stevens and North Carolina State University on a groundbreaking study titled “Magnetic Switching in Monolayer 2D Diluted Magnetic Semiconductors via Spin-to-Spin Conversion.” Published in Advanced Functional Materials in February 2025, the research explores innovative methods to control electromagnetism in ultra-thin materials by spinning electrons in the opposite direction that they do in traditional technology. This work could pave the way for more efficient and compact electronic devices, pushing the boundaries of modern technology.
Modern technology’s reliance on electric charge for data storage limits memory and power efficiency. To address this, the team explored spintronics, using electron spin to manipulate magnetic properties in ultra-thin materials. They developed iron-doped molybdenum disulfide (Fe: MoS ₂), combining molybdenum disulfide (MoS₂), a one-atom-thick semiconductor, with iron to maintain magnetism without external power. Fe: MoS ₂ achieves room-temperature magnetism, eliminating the need for extreme cooling, and enables low-power memory storage by flipping magnetic switches using electron spins. At just one atom thick, 100,000 times thinner than a human hair, it remains stable above room temperature, making it ideal for compact electronics. Additionally, it reduces power consumption by nearly 100 times compared to existing methods, setting a new standard for energy-efficient technology.